Geomatics is the discipline of electronically gathering, storing, processing and delivering spatially related digital information. This broad term applies to both science and technology, and integrates the more specific disciplines and technologies of geodesy, surveying, mapping, positioning, navigation, cartography, remote sensing, photogrammetry and geographic information systems (GIS).
BGS has pioneered the use of ground-based (terrestrial) and near-Earth techniques for a variety of geoscientific applications since 1999. These have been used on a variety of projects such as:
- monitoring actively growing volcanic lava domes
- monitoring rapidly retreating glaciers
- coastal erosion and platform evolution
- inland and coastal landslide modelling
- mapping of geological structures and fault boundaries
- rock stability
- subsidence feature analysis
- creating surrogate outcrop models for oil field reservoir rocks
- geo-conservation projects, such as archiving and analysis of important outcrops, quarries and other temporary exposures
In fact, BGS has carried out over 300 surveys at more than 150 sites, both in the UK and overseas.
Quick links
- Terrestrial LiDAR Scanning
- Unmanned Aerial Systems (UAS)
- Mobile Mapping Solution
- Tracking glaciers’ retreat
- Monitoring shifting coastlines
- Measuring active volcanos
- Case studies & downloadable reports
- TLS locations
- Publications
Terrestrial LiDAR scanning
As a tool of modern geoscience, the use of terrestrial LiDAR scanning allows unprecedented resolution and accuracy.
The BGS uses Riegl and Faro terrestrial light detection and ranging (LiDAR) scanners because of their flexibility, range and portability. We have a very long-range scanner that can make measurements at distances of over 2000 m, a medium-range scanner that can make accurate measurements at distances of up to 800 m and a high-speed short-range (300 m) scanner that can take measurements at a rate of 972000 points/second with an accuracy of ±3 mm.
Our newest model is the Riegl VZ-1000, which can scan up to 1400 m with an accuracy of ± 8mm and a measurement rate of 62000 points/second. A high-resolution digital camera coupled with a Leica global navigation satellite system (GNSS) enables coloured point-clouds, textured triangulated surfaces or orthophotos, with intensity and depth information, to be captured, accurately geo-referenced and processed.
Modern terrestrial LiDAR scanners (TLS) have significantly increased the level of resolution and accuracy achievable, and the speed of data acquisition. The ability to capture and measure changes in geological features with time by repeat surveys has revolutionised ground-based geomatic research.
Unpiloted aerial systems (UAS)
Structure from motion photogrammetry (SfM) has emerged as an alternative viable technology, whether deployed on an unpiloted aerial system (UAS) or otherwise. It is lighter, more compact, cheaper to buy and repair, and has lower power requirements than TLS.
BGS has six multi-rotor UAS, which it uses to collect data for ground surface and geological investigations. UAS are becoming increasingly common for civilian use, with the Chinese manufacturer DJI and the US company Parrot dominating the market. They are used in such fields as:
- geology
- geomorphology
- surveillance
- film making
- scientific research
- surveying
- disaster relief
- law enforcement
- archaeology
- forestry and agriculture
- recreation
Like many other organisations, BGS is able to utilise the usability and portability of these aircraft to create photogrammetric images and point clouds using SfM techniques at a fraction of the cost of using a system mounted on an aeroplane. The resultant data, though not as accurate as that obtained from TLS, should still be considered more than adequate for most uses, and gives the ability to create both 2D and 3D full-colour models.
BGS uses drones to gain a perspective of the Earth that is simply not possible with instruments that are based on the ground. For more information please see our drone research page.
Mobile mapping solution
BGS was one of the first companies in the UK to purchase the Leica Pegasus Backpack, a wearable reality-capture sensor platform. The backpack combines five 4 MP cameras, offering a fully calibrated 360° × 200° view, with two 16-channel LiDAR profilers, capable of scanning 600 000 points/second at a range of 50 m, and a triple-band GNSS and inertial measurement unit (IMU), capable of a positional accuracy of 20 mm, in an ultra-light carbon-fibre chassis weighing 13 kg.
The Pegasus Backpack offers great speed and portability advantages over existing terrestrial LiDAR systems, which can weigh in excess of 30 kg and are extremely bulky to carry. This enables us to carry out 3D mapping of long coastal (or inland) sections, landslides and other unstable surfaces, ice sheets and glaciers, railway lines, roads and other linear features. We can also use the backpack to carry out building information modelling (BIM) using both imagery and point-cloud data to document outdoor, indoor and even underground areas.
Disaster responders will be able to capture data in 3D, on foot, in danger zone areas. Simultaneous localisation and mapping (SLAM) technology and a high-precision IMU ensure the achievement of accurate positioning, even during GNSS outages. An external light-source makes precise scanning of tunnels and cave systems possible. The backpack can also be vehicle mounted for mobile mapping, or pole mounted for lowering into voids or sinkholes. This system allows for faster capture of critical data, leading to better-informed decisions, on-site if necessary.
Tracking glaciers’ retreat
By using a combination of repeat TLS surveys, on-ice based GNSS and ground penetrating radar (GPR), we have been able to see the full picture of glacial retreat for the first time. This work has shown that the margin of the Falljökull glacier, in south-east Iceland, has ceased moving and is now undergoing stagnation. However, field and photographic evidence show that the icefall remains active, feeding ice from the accumulation zone on Öraefajökull to the lower reaches of the glacier. To accommodate this continued forward motion, the upper section of the glacier below the icefall is undergoing intense deformation (folding and thrusting) and, as a result, is being thrust over the lower, immobile section of Falljökull.
This type of behavior has never been described before and could have implications for how other steep, mountain glaciers around the world are responding to changes in the climate.
Monitoring shifting coastlines
We use our laser systems to monitor actively eroding sections of coast around England. The techniques we have developed are ideal for this application, as TLS is particularly suited to measuring vertical cliff sections. Measurements from a plane or satellite measure only the top edge of the cliff, whilst measuring them in the field is often very dangerous. Our technique enables us to take measurements of changes in the whole cliff section, from a safe distance, which can be used to model how internal processes within the cliff slope affect coastal erosion.
Our work at Aldbrough, in North Yorkshire, has shown that the cliff is disappearing at a rate of 3 m a year. This erosion is caused by both landslides and the direct action of the sea crashing against it.
Measuring active volcanoes
There can be few more hazardous situations than that of monitoring a volcanic andesite lava dome for signs of an impending collapse. Partial collapse of a lava dome generates hot, fast-moving, pyroclastic density currents. Monitoring in such circumstances requires measurements to be taken from a distance that minimises the threat from eruption and from asphyxiation by volcanic gases. The method also needs to be rapid, to minimise the time spent by the monitoring team in the hazardous zone.
We have used TLS techniques to monitor the growth of the lava dome on the Soufrière Hills Volcano, on the Caribbean island of Montserrat. In May 2006, our measurements helped to identify the changes in lava dome growth rate that directly preceded a total lava dome collapse.
Case studies and downloadable reports
Here five case studies utilising various different pieces of ground-based geomatic equipment, and their applications are presented:
- Aldborough, Yorkshire
- Hellfire Caves, High Wycombe
- Hollin Hill, Yorkshire
- Permian sandstone
- Virkisjökull Glacier Observatory, Iceland
TLS locations
Terrestrial LiDAR scanning has been carried out on over 170 different sites, both in the UK and overseas. You can download more information about the TLS locations.
Publications
Everest, J, Bradwell, T, Jones, L, and Hughes, L. 2017. The geomorphology of Svínafellsjökull and Virkisjökull-Falljökull, south-east Iceland: a land system of rapid glacial retreat. Journal of Maps, Vol. 13(2), 936–945.
Gibson, A D, Forster, A, Poulton, C V L, Rowlands, K A, Jones, L D, Hobbs, P R N, and Whitworth, M C Z. 2003. An integrated method for terrestrial laser scanning subtle landslide features and their geomorphological setting. Proceedings of the Annual Conference on Scales and Dynamics, 10-12 September 2003, University of Nottingham. (Remote Sensing ane Photogrammetry Society (RSPSoc).)
Glendell, M, McShane, G, Farrow, L, James, M R, Quinton, J, Anderson, K, Evans, M, Benaud, P, Rawlins, B, Morgan, D, Jones, L D, Kirkham, M, Debell, L, Quine, T A, Lark, M, Rickson, J, and Brazier, R. E. 2017. Testing the utility of structure from motion photogrammetry reconstructions using small unmanned aerial vehicles and ground photography to estimate the extent of upland soil erosion. Earth Surface Processes and Landforms Vol. 42(12), 1860–1871.
Hobbs, P R N, Jones, L D, Kirkham, M P, Holyoake, S J, Pennington, C V L, Dashwood, C, Banks, V J, and Reeves, H J. 2019. Establishment of a coastal landslide observatory at Aldbrough, East Riding of Yorkshire, UK. Quarterly Journal of Engineering Geology and Hydrogeology, Vol. 53, 88–100.
Hobbs, P R N, Jones, L D, Kirkham, M P, Pennington, C V L, Morgan, D J R, and Dashwood, C. 2019. Coastal landslide monitoring at Aldbrough, East Riding of Yorkshire, UK. Quarterly Journal of Engineering Geology and Hydrogeology, Vol. 53, 101–116.
Hobbs, P, Gibson, A, Jones, L, Poulton, C, Jenkins, G, Pearson, S, and Freeborough, K. 2010. Monitoring coastal change using terrestrial LiDAR. 117–127 in: Elevation Models for Geoscience. Fleming, C, Marsh, S, and Giles, J (editors). Geological Society of London Special Publications 345. (London: Geological Society.)
Hobbs, P R N, Humphreys, B, Rees, J G, Jones, L D, Gibson, A, Rowlands, K, Hunter, G, and Airey, R. 2002. Monitoring the role of landslides in ‘soft cliff’ coastal recession. In: Proceedings of an International Conference on Instability, Planning and Management, Ventnor, Isle of Wight, UK, 589–600. (Thomas Telford: London.)
Jones, L D. 2014. Measuring our changing Earth. Planet Earth, Autumn, 28–29.
Jones, L.D. 2007. The application of terrestrial LiDAR to volcano monitoring – an example from the Montserrat Volcano Observatory. Civil Engineering Surveyor, November 2007, 21–23. (UK: SURCO Ltd.)
Jones, L.D. 2006. Monitoring landslides in hazardous terrain using terrestrial LiDAR: an example from Montserrat. Quarterly Journal of Engineering Geology and Hydrogeology. Vol. 39, 371–373.
Loughlin, S C, Luckett, R, Ryan, G, Christopher, T, Hards, V, De Angelis, S, Jones, L, and Strutt, M. 2010. An overview of lava dome evolution, dome collapse and cyclicity at Soufrière Hills volcano, Montserrat, 2005–2007. Geophysical Research Letters, Vol. 37(19), L00E16.
Ó Dochartaigh, B, E, MacDonald, A M, Black, A R, Everest, J, Wilson, P, Darling, W G, Jones, L, and Raines, M. 2019. Groundwater–glacier meltwater interaction in proglacial aquifers amid rapid glacier retreat. Hydrology and Earth System Sciences, Vol. 23, 4527–4539.
Phillips, E, Everest, J, Evans, D J, Finlayson, A, Ewertowski, M, Guild, A, and Jones, L D. 2017. Concentrated, ‘pulsed’ axial glacier flow: structural glaciological evidence from Kvíárjökull in SE Iceland. Earth Surface Processes and Landforms, Vol. 42(13), 1901–1922.
Philips, E, Finlayson, A, Bradwell, T, Everest, J, and Jones, L. 2014. Structural evolution triggers a dynamic reduction in active glacier length during rapid retreat: evidence from Falljökull, SE Iceland. Journal of Geophysical Research: Earth Surface, Vol. 119(10), 2194–2208.
Philips, E, Finlayson, A, and Jones, L. 2013. Fracturing, block faulting and moulin development associated with progressive collapse and retreat of a maritime glacier: Falljökull, SE Iceland. Journal of Geophysical Research: Earth Surface, Vol. 118(3), 1545–1561.
Poulton, C V L, Lee, J R, Jones, L D, Hobbs, P R N, and Hall, M. 2006. Preliminary investigation into monitoring coastal erosion using terrestrial laser scanning: case study and geological assessment at Happisburgh, Norfolk, UK. Bulletin of the Geological Society of Norfolk, No. 56, 45–64.
Rowlands, K A, Jones, L D, and Whitworth M. 2003. Landslide laser scanning: a new look at an old problem. Quarterly Journal of Engineering Geology and Hydrogeology, Vol. 36, 155–157.
Rumson, A G, Hallett, S H, and Brewer, T R. 2019. The application of data innovations to geomorphological impact analyses in coastal areas: an East Anglia, UK, case study. Ocean & Coastal Management, Vol. 181, 104875.
Ryan, G, Loughlin, S C, James, M, Jones, L, Calder, E, Christopher, T, Strutt, M, and Wadge, G. 2010. Growth of the lava dome and extrusion rates at Soufrière Hills volcano, Montserrat, West Indies: 2005–2008. Geophysical Research Letters, Vol. 37(19), L00E08.
Tapete, D, Banks, V, Jones, L, Kirkham, M, and Garton, D. Contextualising archaeological models with geological, airborne and terrestrial LiDAR data: the Ice Age landscape in Farndon Fields, Nottinghamshire, UK. Journal of Archaeological Science, Vol. 81, 31–48.
Find out more about our research
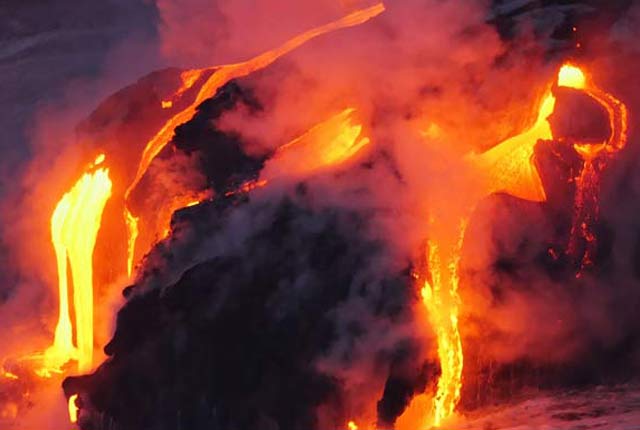
Multi-hazards and resilience
We work with partners worldwide to enhance understanding of hazards, vulnerability, exposure and risk to ensure our science is useful, usable and used.
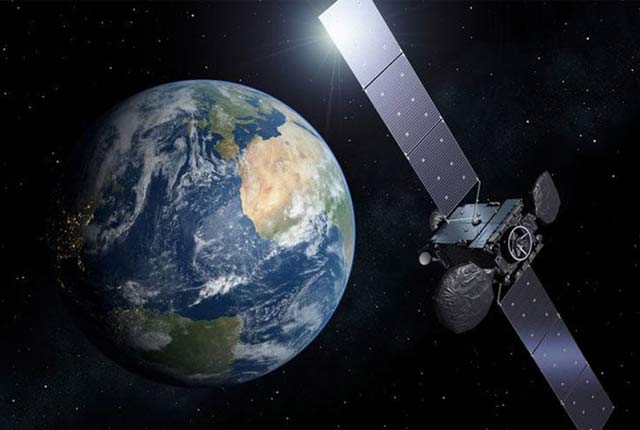
Geodesy and Earth Observation
We develop and use innovative Earth observation (EO) techniques to characterise and monitor Earth processes that impact lives and livelihoods.
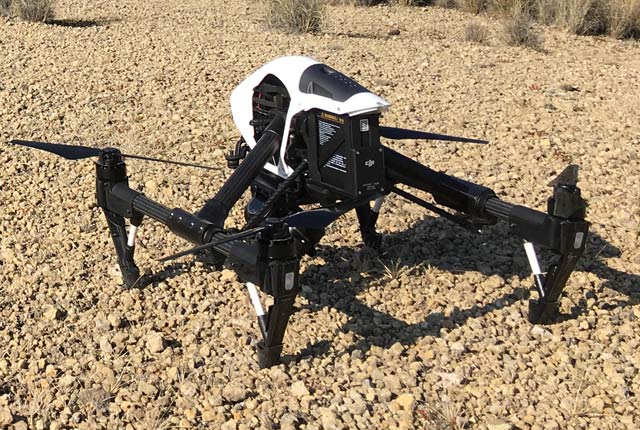
Drone research
BGS use drones to gain a perspective of the Earth that is simply not possible with instruments that are based on the ground.
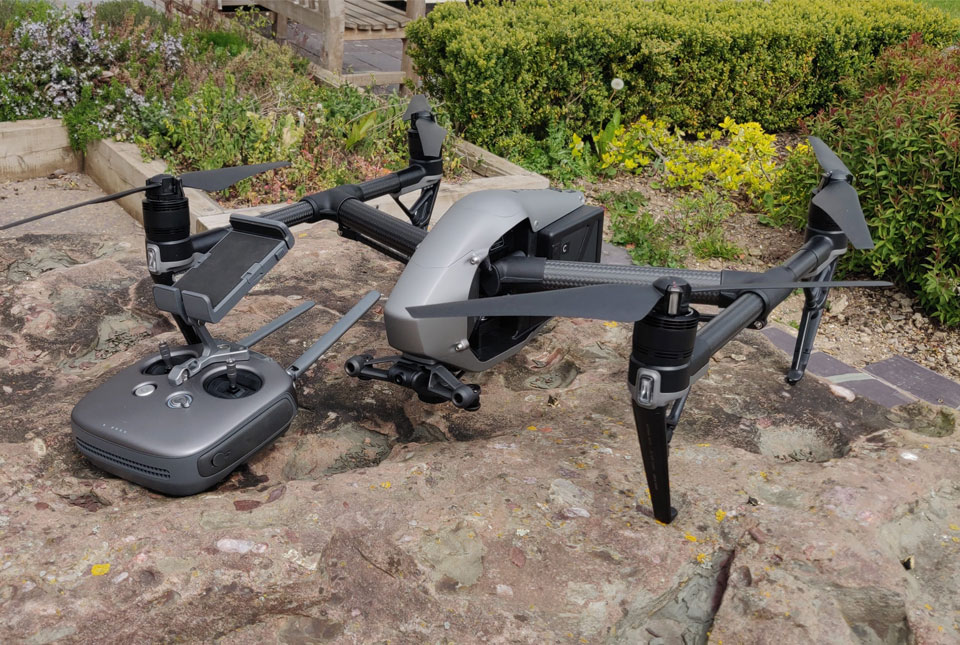
How we use drones
BGS uses unpiloted aerial systems (UAS), or drones, to collect data for geological investigations, mapping and surveying, and for promotional purposes.
Case studies
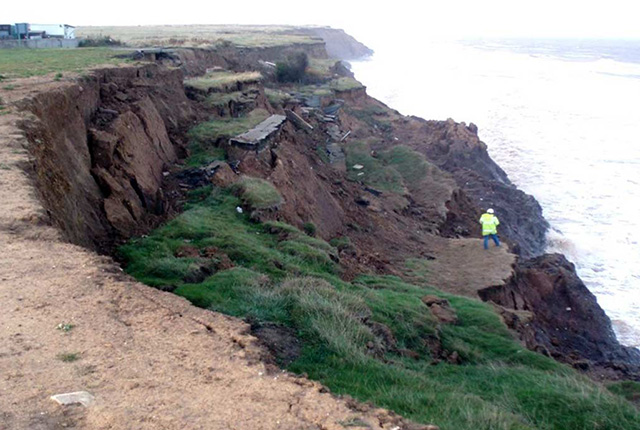
Aldbrough case study
The BGS’s test site at Aldbrough is located on the extensive Holderness coast in South Yorkshire and monitoring the actively receding coastline.
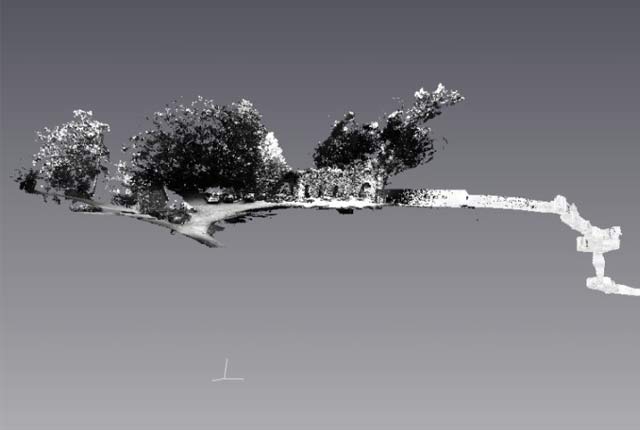
Hellfire Caves case study
In June 2014 the BGS were asked to carry out a terrestrial LiDAR survey of the Hellfire Caves; a network of man-made chalk and flint caverns, extending 500m underground.
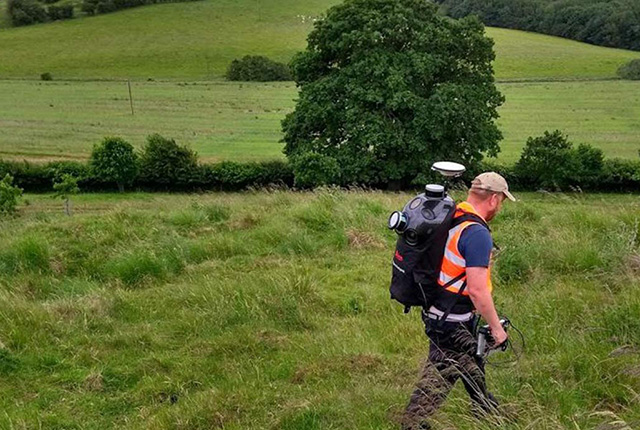
Hollin Hill case study
The field observatory at Hollin Hill brings together a combination of our field surveying, geomorphological, geophysical, engineering and hydrogeological expertise.
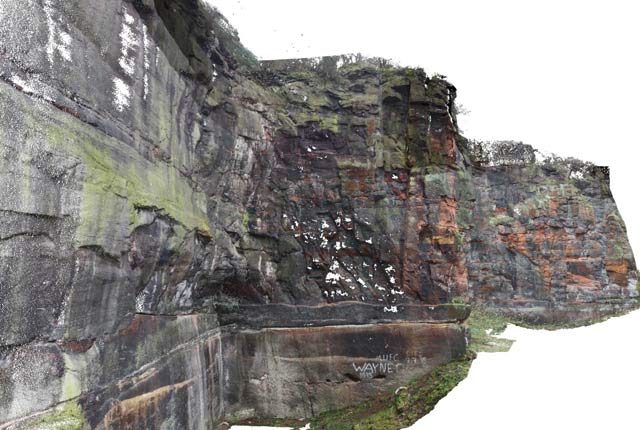
Permian sandstone case study
The main objectives of the Permian Sandstone study are to undertake discontinuity analysis using LiDAR scans and to provide high-quality 3D images for further stratigraphical study.
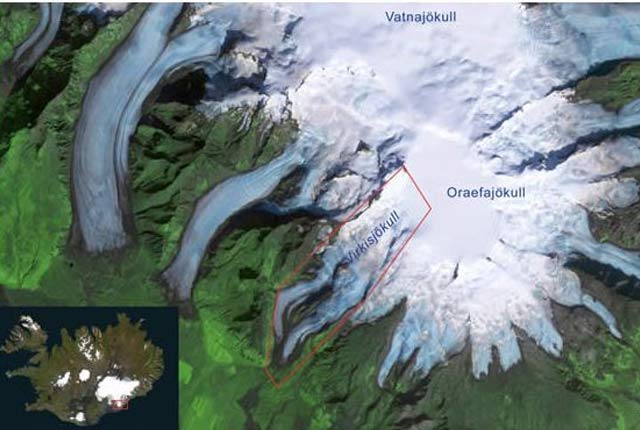
Virkisjökull Glacier Observatory case study
BGS operates an observatory site in south-east Iceland, studying the evolution of the glacier, the surrounding landscape and their responses to regional climate
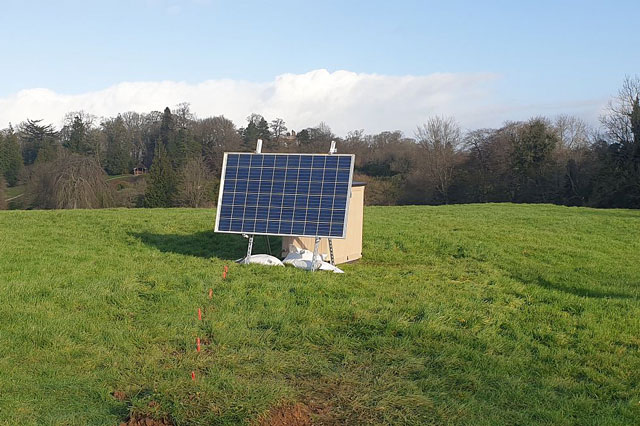
UK’s geomagnetic blind spots tackled with new observatories
28/07/2022
Three new geomagnetic observatories have been installed across the UK to fill in the country’s ‘blind spots’ and tackle the risk posed by space weather.
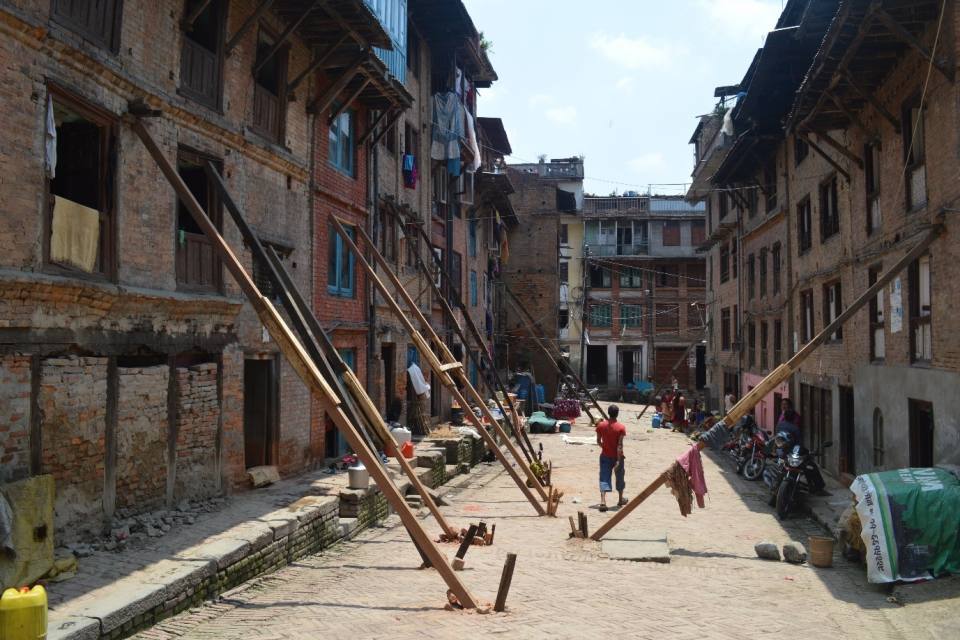
Using satellite imagery for emergency disaster response
06/07/2021
BGS has a long history of assisting relief efforts by providing satellite maps, data and interpretation to those affected by disasters, helping to identify hazardous areas to avoid.
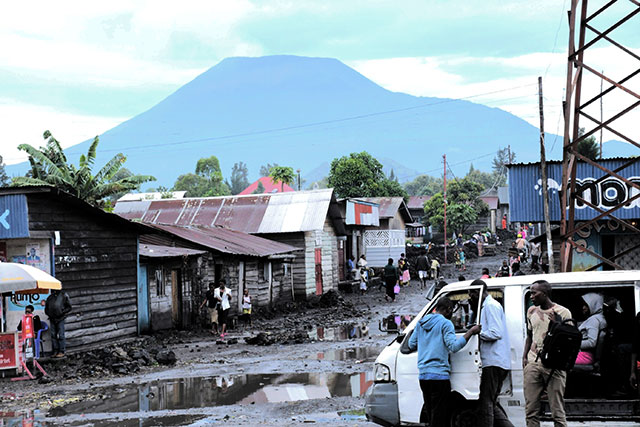
Spaceborne data: an expanding role in disaster response
23/06/2021
Alessandro Novellino explains the importance of Earth observation to help facilitate successful emergency responses after natural hazard occurrences.
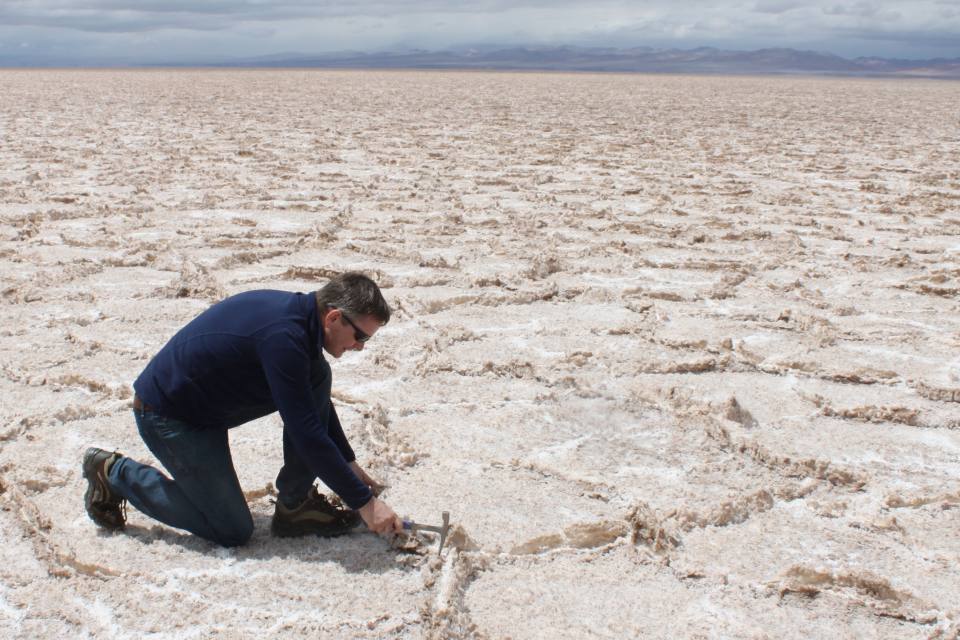
Virtual fieldwork during a global pandemic
03/03/2021
Virtual field reconnaissance can help maintain research momentum during the COVID-19 pandemic.
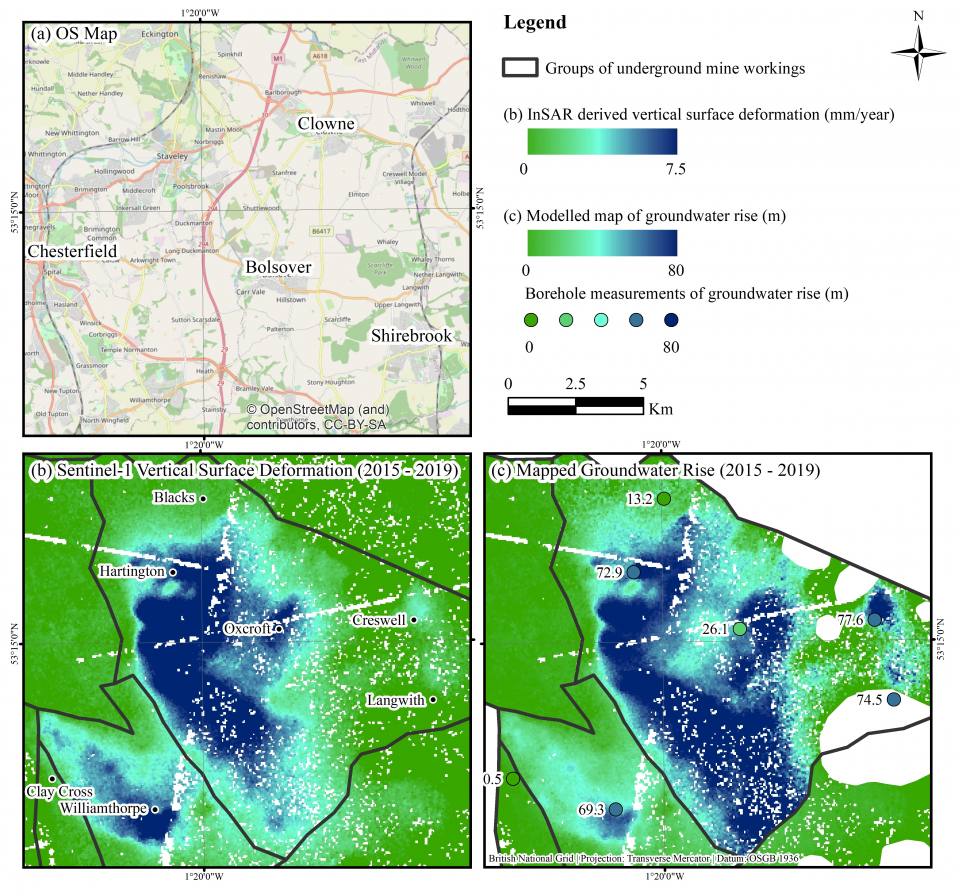
BGS data supports new tool to track geological changes in abandoned coal mines
20/11/2020
BGS data supports new tool to track geological changes in abandoned coal mines.
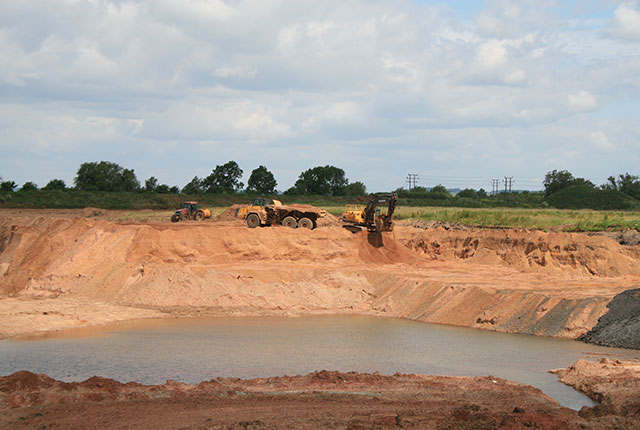
Joint project aims to support sustainable development in Kenya
12/10/2020
BGS is part of a joint UK-Kenyan international project that aims to blend data and information with local stakeholder input to explore the sustainable supply of sand and aggregates in Kenya.
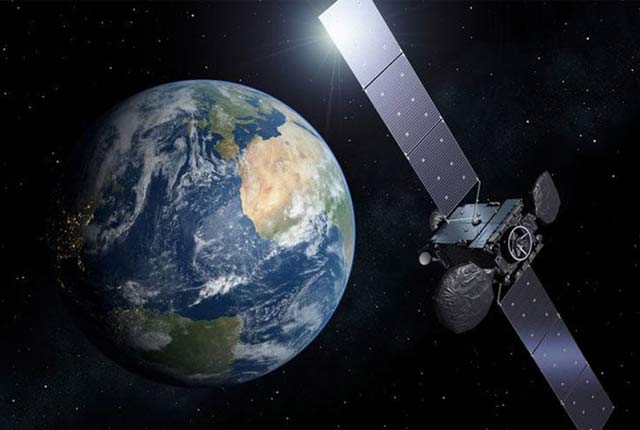
Geodesy and Earth Observation
We develop and use innovative Earth observation (EO) techniques to characterise and monitor Earth processes that impact lives and livelihoods.
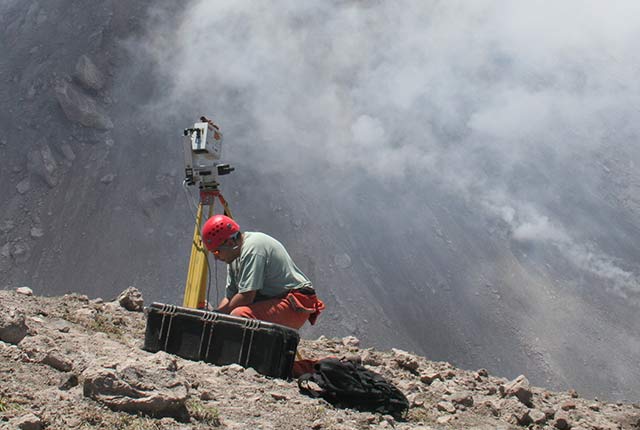
Ground-based and near-Earth geomatic surveys
The BGS has pioneered the use of ground-based (terrestrial) techniques for a variety of geoscientific applications since 1999.
Tsunami sediments study and satellite mapping | Japan fieldwork 2011
24/06/2011
BGS scientists visited north-east Japan to study the area devastated by the tsunami caused by the Great Tohoku earthquake of 11 March 2011.